Hans Peter Broedel
Together with generally good weather and the rapid clearing of arable land, the new modes of farming improved crop yields, leading to rising population. During the high middle ages, Europe’s population doubled, from about 37 million to 74 million, with much of this increase concentrated in the North and West: modern Britain, France, the Netherlands, and Germany.1 The most productive medieval manors were the most specialized; they farmed the most productive crops most intensively. These manors, however, had to trade for everything they could not supply themselves, and required markets in which to sell their surplus. They needed towns. For this reason, although there were only 29 towns with more than 5000 inhabitants in Europe before 1000 CE, by 1200 there were 127.2 Towns generated wealth: they produced cloth, tools, glass and metal ware, furniture, and luxury goods; they provided markets for the sale of grain, fruit, and animals; and they housed butchers, brewers, and specialists of many kinds.
Townsfolk needed very different sorts of technologies than did rural farmers, and they eagerly adopted tools of all sorts when they became available. This happened more frequently than in the early middle ages because the growth of towns and trade in Europe was part of a more general rise in population, agricultural yields and trade that extended all the way from Sung China to West Africa.3 As Europeans became increasingly engaged in this emerging global economy, they gained access to a host of technologies developed in other cultures, and proved adept at adapting them to their own particular needs. One development particularly important for the future was the effort to replace the work of humans and animals with alternative sources of power, a trend particularly noticeable in the spread of mills. Mills are technically machines for grinding or cutting, but when we talk of medieval mills we usually are talking more generally about machines that convert the work of humans, animals, wind or water into rotary power, most commonly to grind grain, but also for many other purposes. Most medieval rivers and streams boasted at least a few mills, and in some places they were extremely common. They were built for the usual reason: they were profitable. In return for an admittedly significant capital investment, mill owners could exploit a resource that was basically free instead of relying on power sources that had to be fed, or, worse, paid. Manor lords were particularly fond of watermills, because lords were able to require their tenants to pay to use them; peasants caught illicitly grinding their own grain at home were liable to pay heavy fines.4
Watermills, of course, had been in use for centuries, but growing wealth encouraged their spread; and more remarkable were the simultaneous efforts to use waterpower in other industries. A key enabling invention was the cam, an irregularly shaped wheel or cylinder that, when mounted on a shaft, can convert rotary to linear motion and vice versa. Cam shafts were known and used in the East and in the ancient world, but whether Europeans inherited them from the Romans or encountered them in Asia is unknown, but by the year 1000, Europeans were adapting them to existing mill technology to power hammers that forged iron and pounded wool into cloth. Soon they were also adapted to make paper, saw wood and stone, and tan animal hides.5 Adam Robert Lucas, a historian specializing medieval technology, determined that medieval mills were employed in the production of at least seventeen different products, ranging from malted grain to metal to opium.6
Like heavy plows and horse collars, however, it should be noted that watermills are similarly just one part of interrelated “technological complex.” To build an efficient overshot watermill requires a number of related skills: water must be channeled into a millpond, and then released through sluice gates into a downward running millrace so that the water pours over the top of a waterwheel providing rotary power.
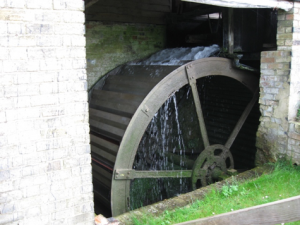
Europeans first developed the necessary hydrological expertise while draining swamps and marshes to gain arable land. For this reason, the best hydraulic engineers were probably the Dutch. Ironically, however, the Netherlands are not only notoriously boggy, they are also famously flat, and suitable streams for mill building were few and far between. A solution to this lack of waterpower appeared by the early twelfth century, when someone had the idea to use the wind as an alternative source of power. Like watermills, windmills had been used for centuries throughout the world, but the European windmill again adapted existing technologies to local conditions in unique ways. The European windmill was extremely large, with massive vertically mounted sails, and was mounted on a post or pivoting cap, that permitted the apparatus to rotate into the prevailing wind. The first windmills were used to grind grain, but they were soon adapted to pump water and drain bogs. By the end of the middle ages, windmills were in use all across Northern Europe to drain marshes and to mill paper, grind corn, turn saw blades and so forth.7
All of this activity can create a false impression of a “medieval industrial revolution.”8 The reality, however, was much more complex. Although in some places Europeans embraced new technologies and deployed them in innovative and sometimes spectacular ways, most did not or did so only seldom. The problem was that to deploy mill technologies on a large scale profitably required an unusual set of conditions: mills were expensive, so abundant capital was required, as was the presence of specialists to build, operate, and maintain the mills. Once built, mills needed plenty of raw materials—grain, , wood, stone, or whatever—to process, and they needed markets in which their products could be sold profitably. And, of course, they required ready and reliable supplies of wind and water. These conditions were found in a few French river valleys, in Northern Italy, and parts of the Low Countries, but seldom elsewhere. As Lucas remarks, the evidence indicates “those regions of medieval Europe that were engaged in industrial milling appear to have been geographical pockets of technological innovation within a broader environment of technological incrementalism.”9 This should not be seen as an insult to medieval Europeans, but as a reminder that we should be careful when thinking about historical change in terms of revolutions and breakthroughs: changes indeed are sometimes “revolutionary,” but far more often they are gradual and incremental.
A second technology with major implications for the future involved time keeping and, curiously enough, its story begins, like watermills, with hydraulic engineering. Humans, of course, have been interested in keeping track of time for all sorts of reasons for just about as long as modern humans have been around, but medieval clergy were particularly fixated on this problem. First, the Christian calendar was unusually complex because to calculate the annual date of Easter, you had to reconcile the Lunar Hebrew calendar with the Solar Julian. This was a source of endless controversy, and is so complicated that it has never been resolved to everyone’s satisfaction, which is why even today Eastern and Western Christians celebrate Easter on different days. Second, the duties of Benedictine monks were regulated according to the hours of the day, which were announced by the ringing of a bell (our English word “clock” comes from the Latin, clocca, meaning “bell”). For these reasons the clergy were keenly interested in keeping time. Sun dials were a possibility, but were not much use on cloudy days and tracking time by just keeping an eye on the sun suffered from the same problem. Hence, at least by the tenth century, some monasteries were using water clocks to keep track of the hours of the day.10
Water clocks, also known as clepsydra (water-thief), had been used since antiquity, and medieval Europeans had access to the fine description of their operation in the De architectura (On Architecture) of the Roman military engineer, Marcus Vitruvius (fl. 25 BCE).11 In this clock, water flowed at a controlled rate from a water source into a tank. As the water rose, it lifted a float, and this motion could be used to turn the dial of a clock face or release weights to ring bells. The Abbasid Caliph in Baghdad, Harun al-Rashid, had sent a very elaborate and elegant clepsydra as a gift to Charlemagne, complete with twelve mechanical horsemen that would ride out on the hour.12 We can imagine that the Frankish king was duly impressed, but simpler versions of these early alarm clocks seem to have been fairly common monastic conveniences.13 Yet water clocks had obvious disadvantages as well: they were inaccurate and fiddly; in the summer, the water in their tanks evaporated, and in the winter, it would freeze.
Yet the first mechanical clock was almost certainly not invented merely to tell time but rather to do something far more complicated, to model the motion of the stars and planets. This is not as strange as it might appear in this age of digital time, for all traditional analog clocks are just devices which model the path of the sun as it moves across the sky.14 Since antiquity, however, astronomers and horological engineers (“horology” is the study of time) had been inventing devices to model more complex celestial relationships. Islamic scholars were particularly good at this because of the complexity of their lunar calendar, and their need to determine latitudes to find the direction of Mecca, and the time for morning prayers. For similar reasons, European clergy were interested in heavenly motion and eagerly learned everything they could from Muslim texts and exemplars. The astrolabe, a device used to determine the position of stars and planets and to work out time and latitude, was almost certainly introduced to medieval Europe from Muslim Spain.15
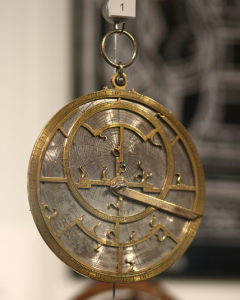
As in many similar cases, however, Europeans applied this inherited expertise to their own problems in new ways, and just as the camshaft enabled them to devise new uses for mills, the escapement proved the essential technological component of the mechanical clock.
An escapement is a gear that restricts the rotation of another gear or shaft so that it moves only in certain well defined increments; sometimes a pendulum regulates the speed of rotation, but there are a number of alternatives. In all cases, it meant that hanging weights suspended from a cord wound about a shaft could be used to drive mechanical clocks, as could a coiled spring. Who invented the first escapement is a mystery, but records of clocks probably using them begin to appear in Europe around the close of the thirteenth century.
Then in the fourteenth, an English monk, Richard of Wallingford, wrote a detailed description of his extremely complex astronomical clock: not only did his clock tell time, but also modeled the motion of the sun and moon, it predicted solar and lunar eclipses, and calculated the times of high and low tides.16
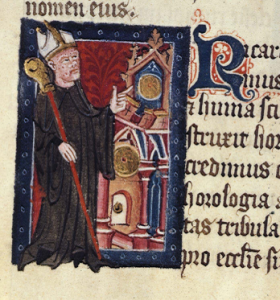
This was a legitimate marvel of engineering, requiring extremely complex calculations to be performed mechanically through the timed intersection of meticulously crafted gears. It also illustrates how sometimes in history a very complicated invention precedes a simpler one. For, although astronomical clocks were of great value and interest to the church and its scholars, much simpler mechanical clocks, primarily used just to tell time, sprang up in European towns and cities almost immediately.
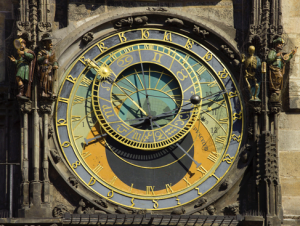
Already by the mid-fourteenth century, the hours were being counted on a Paduan city clock from noon and midnight, as we do now.17
The regular chiming of bells permitted, or perhaps better, required that people use time to regulate their lives. Now for the first time you could meet for lunch at one o’clock and reasonably expect your associates to be on time. Now the workday would run to ten or twelve equal hours, regardless of the time of year. More importantly, perhaps, the spread of clocks made time public and secular, and not under the control of the church. In later years, this understanding of secular time would play a pivotal role in the development of science, business, and urban society.
1 Moore, 30; Kay Slocum, Medieval Civilization (Belmont, CA: Thomas Wadsworth, 2005), 136.
2 Moore, 31.
3 William Thompson, The Emergence of the Global Political Economy (London: Routledge, 2000).
4Jean Gimpel, The Medieval Machine: The Industrial Revolution of the Middle Ages (New York: Penguin Books, 1977), 12-15.
5 Frances Gies and Joseph Gies, Cathedral, Forge, and Water-Wheel: Technology and Invention in the Middle Ages (New York: Harper Collins, 1994), 115-117; Arnold Pacey, The Maze of Ingenuity: Ideas and Idealism in the Development of Technology , 2nd ed. (Cambridge MA: MIT Press, 1992), 12.
6 Adam Robert Lucas, “Industrial Milling in the Ancient and Medieval Worlds: A Survey of the Evidence for an Industrial Revolution in Medieval Europe,” Technology and Culture 46.1 (2005): 1-30; 14.
7 Dyer, 299; Michael Pye, The Edge of the World: A Cultural History of the North Sea and the Transformation of Europe (New York: Pegasus Books, 2015), 258-260.
8 Lynn White, Medieval Technology and Social Change (London: 1968), 89; Gimpel expresses this argument even more forcefully: The Medieval Machine: The Industrial Revolution of the Middle Ages.
9 Lucas, 29.
10 This and subsequent paragraphs are deeply indebted to John North’s terrific book, God’s Clockmaker: Richard of Wallingford and the Invention of Time (London: Continuum, 2005).
11 North, 151.
12 E.A. Truitt, Medieval Robots: Mechanism, Magic, Nature, and Art (Philadelphia: University of Pennsylvania Press, 2015), 21.
13 North, 148.
14 Pacey, 35-43.
15 James Hannam, The Genesis of Science: How the Christian Middle Ages Launched the Scientific Revolution (Washington D.C.: Regnery, 2011), 21.
16 North, 201-218.
17 Gimpel, 164.
18 An idea explored in Jacques Le Goff, “Merchant’s Time and Church’s Time,” in Time, Work, and Culture in the Middle Ages, Arthur Goldhammer, trans. (Chicago: University of Chicago Press, 1982), 29-42.